by Mark Howard, Managing Director, Zettlex
Direct drives differ from traditional drive arrangements. These motors impart many design advantages, but perform best with specialty feedback.
Direct-drive motors have been around for a while, but only recently have OEMs and system integrators begun to leverage the advantages of this technology. Over the last few years, there’s been a huge increase in the number of applications using direct-drive motors. As we’ll explore, some types perform best when designers fit them with a feedback device called an incoder.
Basic direct-drive terminology
The term direct drive can apply to any motor that directly drives a load or rotor without mechanical power-transmission components such as gears, pulleys or chains. Usually though, the term refers to brushless permanent-magnet motors—synchronous motors—that transmit torque directly to the load or rotor. Often, axial height is shorter than the diameter; many versions also sport a large through-bore in their centers.
Another common term for direct-drive motors that produce a constant torque when stationary or moving over small angles is torque motor. Depending on the version, other direct-drive motors are sometimes called Lorenz-force motors and pancake motors.
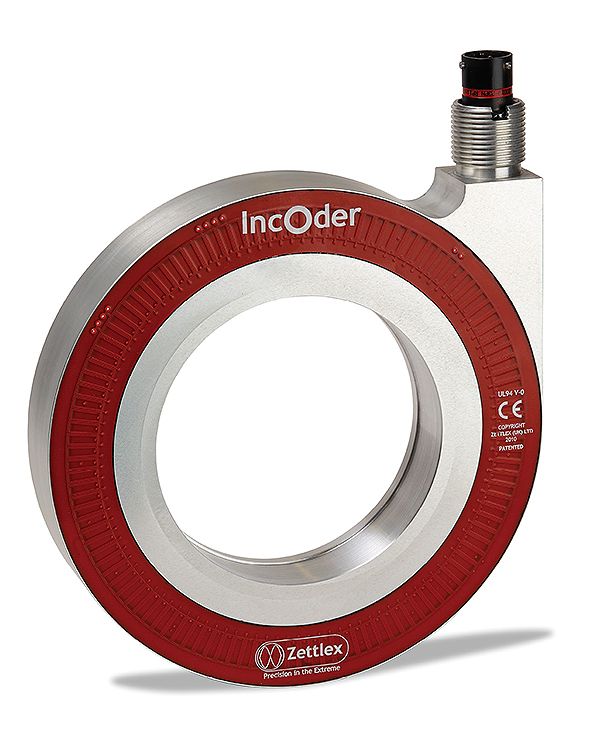
How direct-drive motors work
Direct-drive motors work much like other brushless dc motors. Magnets on the motor’s rotor and windings on the motor’s stator interact when a power supply energizes the windings. That’s when the windings produce electromagnetic fields that attract or repel the rotor’s magnets. Precise commutation of power to the windings spurs controlled motion. Both rotary and linear direct-drive motors exist, but rotary types are most common.
Direct-drive motors usually have many poles—more than 30 and sometimes more than 100. This lets them output high torque at no or low speed, usually slower than 1,000 rpm. Direct-drive motors with diameters of more than 3 ft (1 m) can produce torque of more than 10,000 Nm.
Many direct-drive motors are frameless. Manufacturers sell these without bearings, housing, or feedback sensors. Such products let machine builders and system integrators streamline housing, bearing and shaft design to optimize machine weight, size, shape and dynamic performance.
Direct-drive motors have higher torque-to-inertia ratios than traditional motor arrangements, and they have a low electrical time constant. So, the motors promptly output torque when supplies feed voltage to the windings—getting what control engineers call “good servo stiffness.” More traditional motors generate maximum torque at higher speeds, typically at speeds greater than 1,000 rpm, and engineers size and specify them on their power rating. In contrast, engineers usually size and select direct-drive motors by maximum or continuous torque instead of power.

Advantages and disadvantages
Direct drives offer excellent dynamic performance and accurate control of position and speed. They are reliable and exhibit no backlash or wear thanks to their low part count and elimination of gears, pulleys, seals and bearings. In addition, they offer:
• low torque ripple or cogging
• low acoustic noise and minimal self-induced vibration
• compactness, with low axial height and large bore
• high torque-to-inertia ratio and high torque-to-mass ratio; high torque at low speeds
• energy efficiency thanks to elimination of intermediate mechanical friction and inertia
• relatively large airgaps to resist shock and survive dirty environments
• minimal cooling requirements due to advantageous thermal geometry<
Design engineers choose direct drives for their dynamic performance and shape. The latter is relevant when the motor takes a flat form with a big hole in the middle to let slip rings, pipes and cables pass through.
The main disadvantage of direct-drive motors—that of high cost—is often exaggerated. Direct-drive motors are more expensive than traditional motors in a simple 1:1 comparison. However, cost analysis that accounts for the savings in omitting intermediate gears and couplings (and associated maintenance) and overall mechanical simplification often finds direct-drive arrangements the most cost effective option.
What’s more, the cost premium for direct-drive motors is coming down as direct drives are increasingly common, and there’s more availability of powerful neodymium-iron-boron (Nd-Fe-B) magnets. Case in point: The current cost-to-performance point of direct-drive motors is making them increasingly common in cost-sensitive applications such as washing machines. Here, engineers are replacing traditional motor-and-belt-and-pulley systems with quieter and more reliable direct-drive motors.
More classic examples of direct-drive applications are gimbals in antenna systems (such as vehicle mounted satellite communications), electro-optics, scanners, rate tables, telescopes, surveillance and CCTV cameras, radar and weapons systems. There are also applications in packaging equipment, CNC machine tools, robotics and even high-end record turntables.
Most traditional motors exhibit positional torque ripple known as cogging. At high speeds, this effect is usually inconsequential, as the frequency is so high that it has negligible impact on performance. Direct-drive units suffer more from this phenomenon unless the motor controls use feedback to counteract the effect. One factor that may have slowed the uptake of direct-drive motors is that they need precise electrical control. Only in recent years have sufficiently responsive and fast controllers with update rates of more than 4 kHz become widely available at realistic costs.
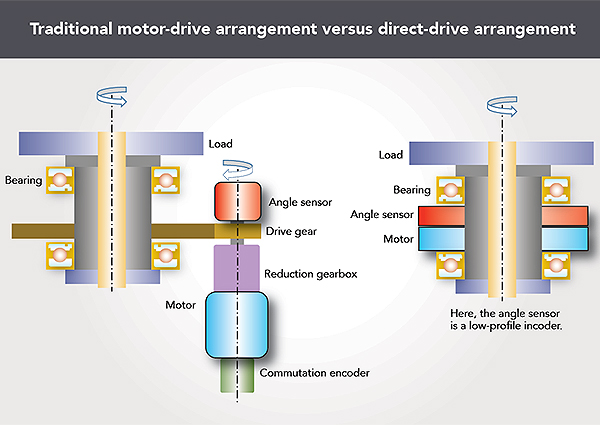
High-resolution position feedback hard to find
A major advantage of direct-drive motors is increased positional, speed, and dynamic accuracy. Direct-drive motors attach directly to the load so there’s no hysteresis, backlash or lost motion in any direction of movement. The only caveat is that they need high-resolution position feedback to complete the servo loop. Sometimes designers use Hall-effect sensors for commutation of power to the motor’s windings. But other feedback is necessary where this setup is insufficient to get precise position or speed control.
If the direct-drive bore is fairly small, say less than 2 in. (50 mm), there are several position-feedback options based on optical, magnetic, capacitive and inductive sensing. However, many sensors don’t have the right morphology for larger motors. Remember that key factors for choosing a direct drive are form factor and a large through bore of more than 2 in. Because most position sensors are for installation on a small input shaft or small through bore, options for suitable position sensors for direct drives have, until recently, been limited.
Options for precise position or speed control
One option is optical ring encoders. These run off a simple dc input and then output absolute or incremental digital signals. Unfortunately, they’re unsuitable for dirty or wet environments because the optical sensor’s path must stay clear. These also don’t withstand extreme temperatures or shock and need accurate installation to get good measurements. Capacitive encoders present similar issues and complicate installations due to their need for dissipation of static-charge buildup on the rotor.
Another option is magnetic-ring encoders. Their advantage over commutation-tracking Hall-effect sensors is modest, as magnetic hysteresis limits their precision. Because they use sensing of dc magnetic fields, they are also susceptible to stray magnetic fields from the motor.
The third and most common option is a brushless resolver. Resolvers use electromagnetic physics, much like the motor itself, to sense rotor position relative to the stator. Debris doesn’t affect resolvers; they have an unrivalled reputation for ruggedness, reliability and safety.
For this reason, resolvers are the standard choice in many high-reliability and safety-related applications, especially in aerospace and defense. But resolvers can also be bulky, heavy and expensive, especially in large-bore formats called pancake or slab resolvers. In fact, the use of these resolvers is probably responsible for the misperception that direct-drive systems are overly expensive for some applications.
New option: Inductive encoder
Now, a new type of sensor delivers direct-drive position feedback. Called inductive encoders, or incoders, these are new-generation inductive sensors that work like traditional inductive sensors for noncontact measurement. But instead of bulky spools of wire, these sensors use printed circuits on flexible or rigid substrates.
Such incoders greatly reduce production cost, size and weight. They offer greater form-factor flexibility, too. There aren’t any sources of inaccuracy from the winding process. They are capable of complex measurement geometries such as curvilinear, 2D and 3D position sensing. In addition, multiple sensors can work in the same space thanks to multi-layer circuit boards (to function as redundant sensors in safety-related applications).
EMC performance is as good as that of resolvers or LVDTs. In fact, incoders are already going into aerospace and military applications.
One catch is that incoders are more expensive than potentiometers.
However, incoders excel because they don’t need the complex ac supply and signal processing that resolvers need. Instead, incoders use simple electrical interfaces similar to those offered by optical encoders. So they take dc power input and output digital electronic signals. Incoders come in absolute or incremental (A/B pulse) formats with resolutions to about 4 million counts per revolution and accuracies better than 40 arc-sec.
Until recently, incoders were too slow for dynamic applications, but new versions offer update rates to 10 kHz. In addition, frameless formats without bearings or couplings can mechanically fix to the machine.
Zettlex
www.zettlex.com
Leave a Reply
You must be logged in to post a comment.