Solid surfaces are subjected to two types of friction: static friction and kinetic friction. Static friction acts when the surfaces are stationary — think of a box on the floor. Static friction is what keeps the box from moving without being pushed, and it must be overcome with a sufficient opposing force before the box will move. Kinetic friction (also referred to as dynamic friction) is the force that resists the relative movement of the surfaces once they’re in motion.
The static friction between two surfaces is always higher than the kinetic friction (at least, in practical, real-world applications). But why is this? To find out, let’s look at the causes behind each type of friction.
Static friction
There are several theories regarding the causes of static friction, and like most friction-related concepts, each one proves valid under some conditions, but fails under other circumstances. For real-world applications (especially those related to industrial machinery and motion control) the two most widely-accepted theories behind static friction have to do with the microscopic roughness of surfaces.

Regardless of how “perfectly” a surface is machined, finished, and cleaned, it will inevitably have asperities — essentially “roughness,” consisting of peaks and valleys, much like a mountain range. (Technically the “peaks” are the asperities.) When two surfaces are in contact, it may appear that they have a large, well-defined area of contact, but in reality, contact occurs only at certain places — that is, where the asperities of both surfaces interfere. The sum of these small areas of contact between the asperities is referred to as the “real” or “effective” area of contact.
Because these individual areas of contact are very small, the pressure (pressure = force ÷ area) between the surfaces at these points is very high. This extreme pressure allows adhesion to occur between the surfaces, via a process known as cold welding, which occurs at the molecular level. Before the surfaces can move relative to each other, the bonds that cause this adhesion must be broken.
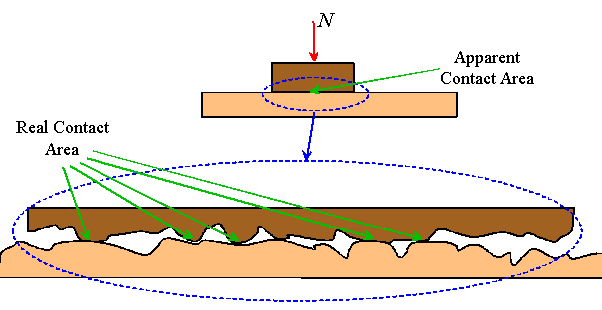
In addition, the roughness of the surfaces means that at some locations, the asperities of one surface will settle into the valleys of the other surface — in other words, the surfaces will interlock. These interlocked areas must be broken or plastically deformed before the surfaces can move. In other words, abrasion must occur.
So, in most applications, static friction is caused by both adhesion and abrasion of the contacting surfaces.
Kinetic friction
Overcoming the static friction between two surfaces essentially removes both the molecular obstacles (cold welding between asperities) and, to some degree, the mechanical obstacles (interference between the asperities and valleys of the surfaces) to movement. Once movement is initiated, some abrasion continues to occur, but at a much lower level than during static friction. And the relative velocity between the surfaces provides insufficient time for additional cold welding to occur (except in the case of extremely low velocity).
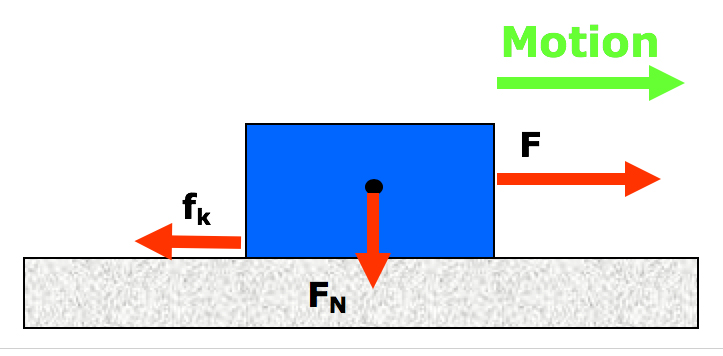
With most of the adhesion and abrasion being overcome to induce movement, the resistance to motion between the surfaces is reduced, and the surfaces are now moving under the influence of kinetic friction, which is much lower than static friction.
Assumptions regarding friction
Friction is an incredibly complex force that manifests differently under various conditions, making it difficult to express in terms of physical laws and mathematic equations. However, there are three assumptions regarding friction that apply in most real-world situations:
- The frictional force is proportional to the normal force.
- The frictional force is independent of the apparent area of contact.
- The frictional force is independent of the relative velocity.
You might notice that assumption #2 (friction is independent of area of contact) seems to contradict the idea presented earlier that because the points of contact between the asperities are very small, pressure between the surfaces is high and adhesion (via cold welding) occurs, which increases friction. But notice that assumption #2 specifies the “apparent” area of contact.
Using the box example from above: Assume you move the contents of the box to a different box with a larger footprint. The mass (and hence, the normal force) hasn’t changed, but the apparent area of contact is larger. Despite the larger apparent area of contact, the friction force remains the same, as predicted by assumption #2.
Why does rolling motion experience friction?
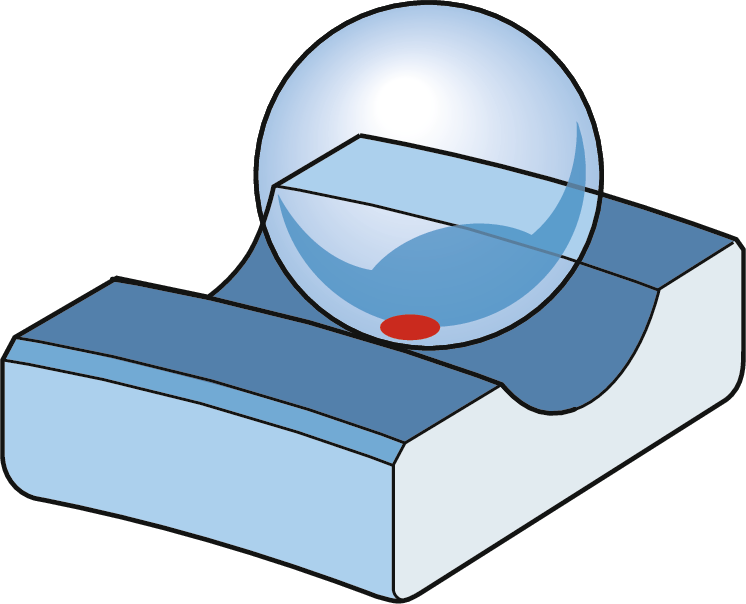
Image credit: SKF
Note that the discussion above relates to non-lubricated, sliding surfaces. In theory, rolling surfaces, such as those found in most rotary and linear bearings (except plain bearings), should not encounter friction forces. But in real-world applications, three factors cause friction in rolling surfaces:
- Micro-slip between the surfaces (the surfaces slide relative to one another)
- Inelastic properties (i.e. deformation) of the materials
- Roughness of the surfaces
Leave a Reply
You must be logged in to post a comment.